Health
Post-Acute Effect of SARS-CoV-2 Infection on the Cardiac Autonomic Fun
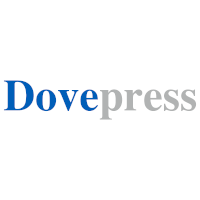
Introduction
SARS-CoV-2 (COVID-19) infection was first reported in China in late December 2019. It has quickly escalated to become a global pandemic causing a catastrophic effect on the world. Cases are increasing all around the world, and the number of people infected reached hundreds of millions, with about 6 million deaths in the first quarter of 2022 worldwide.1,2
Recently, many reports showed a long-term effect of COVID infection that could extend beyond the active disease and the respiratory system. Disturbance in sleep, concentration impairment, fatigue, and palpitations are part of the long-lasting effect of COVID-19 (also known as LONG COVID).3 Post-COVID-19 syndrome is a group of symptoms that affect various body systems after being acutely infected by COVID-19. The symptoms can last longer than 12 weeks after COVID-19 infection, which cannot otherwise be explained alternatively.4 The development of post-COVID-19 syndrome is higher following severe acute illness, but it may develop after mild and moderate acute COVID-19.5,6
A wide spectrum of body dysfunctions has been linked to the chronic effect of COVID-19 infection, including disturbed lung function, endothelitis, thromboembolism, kidney failure, gastrointestinal impairment, mood changes, cognitive disturbances, and hyperglycemia without diabetes mellitus.7 Cardiovascular complication such as myocardial ischemia, infarction, myocarditis, and cardiac arrhythmias are noticeable sequelae of COVID-19 infection, with different suggested pathophysiological mechanisms involving direct damage to the circulatory system due to binding of viruses to angiotensin-converting-enzyme 2 receptors (ACE2), and systemic inflammation.8 However, the consequence of COVID-19 infection on the autonomic regulation of the heart remains unclear.
The autonomic nervous system (ANS) plays a key role in the regulation of the cardiac rhythm.9 Heart rate variability (HRV), cardiovascular autonomic reflex test (CART), and baroreceptor sensitivity (BRS) are non-invasive assessment tools for the autonomic nervous system functions.10,11 Specifically, HRV aids in the evaluation of the sympathetic and parasympathetic functions on the cardiovascular system. Therefore, reflecting dysautonomia and sympathovagal balance.12
Dysautonomia is commonly recognized as a failure in the functions of the autonomic nervous system that can include various symptoms and signs such as fatigue, postural hypotension, changes in blood pressure, arrhythmias, and bladder and bowel function impairment.13 Dysautonomia following viral infections is not uncommon; many viral infections could cause dysautonomia including HIV, mumps, EBV, HBV as well as Coxsackie B virus.14 Recent reports link dysautonomia with COVID-19 infection.15 Involvement of the nervous system occurs probably by direct viral invasion, synaptic spread, or through the blood. Additionally, immunological damage, vascular damage, and hypoxia due to COVID-19 pneumonia, are proposed pathogenic mechanisms for COVID-19 neurological manifestations.16
Orthostatic hypotension (OH) and postural tachycardia syndrome (POTS) have been reported in the post-acute phase of COVID-19 infection.17 Another recent questionnaire-based cross-sectional study found that post-COVID autonomic disturbances are mostly manifested as orthostatic hypotension, gastrointestinal disturbances, and secretomotor abnormalities.18 Additionally, Adler et al reported a reduction in the HRV among post-COVID patients 3 and 6 months after recovery, which may increase the cardiovascular risk among post-COVID survivors.19 In contrast, parasympathetic overactivity with increased HRV was found after 12 weeks from the acute COVID-19 infection.20 Cardiovascular dysautonomia was also detected in about 15% of recently recovered COVID-19 patients (within 30–45 days), with a significantly lower HRV compared to healthy controls.21 Autonomic nervous system dysfunction has also been revealed during the early phase of SARS-CoV-2 infection, with a significant reduction in HRV, BRS, and high incidence of orthostatic hypotension, indicating significant cardiovascular risk.22
However, there is a paucity of research on the chronic sequelae of COVID-19 infection on cardiac ANS functions. Thus the current study aimed to evaluate the post-acute impact of COVID-19 infection on cardiac autonomic nervous system functions, using cardiovascular reflex tests (CARTs), heart rate variability (HRV), and cardiac baroreceptor sensitivity (cBRS).
Methods
This was a comparative cross-sectional observational study carried out in the physiology department’s laboratories at Imam Abdulrahman Bin Faisal University (IAU), College of Medicine, Saudi Arabia, in the period between November 7, 2021, and March 14, 2022. The study population was divided into two groups: controls (n=31) who neither tested positive nor had a history of COVID-19 before, and post-COVID patients (n=28) who tested positive PCR for COVID-19 at least 3 months before recruitment. We determined the sample size based on previous studies with comparable outcomes, where the sample size ranged from 2519 to 15222 participants.
Confirmation of COVID infection is based on positive testing of SARS-CoV-2 unique viral sequencing by using real-time reverse-transcription polymerase chain reaction (rRT-PCR).23
Subjects were excluded if they had: severe acute illness needing hospitalization, nervous system disorders (eg, multiple sclerosis, Parkinsonism, polyneuropathy, and Guillain-Barré syndrome), heart disease (eg, valvular heart disease, cardiomyopathy, arrhythmia, ischemic or congestive diseases), alcoholism, liver disease, malignancies, inflammatory diseases, renal diseases, or taking anti-hypertensive treatments.
Over the recruitment period, the medical records of COVID-19 patients in King Fahad University Hospital (KFUH) were reviewed and those fulfilling the inclusion criteria were contacted to do the autonomic function tests in our physiology laboratory.
The study followed the principles of the Declaration of Helsinki,24 and was approved by the Institutional Review Board of Imam Abdulrahman Bin Faisal University (IRB-UGS-2021-01-391). Informed written consent was obtained from every participant.
Parameters and Procedures
Experimental data was obtained by measuring (1) the baseline cardiovascular autonomic activity through heart rate variability (HRV), (2) cardiovascular reactivity through cardiovascular reflex tests (CARTs), and (3) cardiac baroreceptor reflex sensitivity through determination of baroreceptor sensitivity (cBRS).
Assessment of Baseline Autonomic Activity through Heart Rate Variability (HRV)
After an initial rest of 5 minutes in a supine position on a tilt table, resting HR and BP were measured with SPOT vital sign monitor (NY 13153). The subjects were properly strapped to the tilt table and hooked up to an 8 channel Powerlab 8/35 system (ADInstrumennts, Australia) for continuous recording of ECG, respiratory rate and finger arterial blood pressure. Single lead ECG was recorded by attaching two ECG electrodes on both shoulders through ECG box and bio-amplifier (ADInstruments, Australia). Respiratory rate was monitored through the respiratory belt (ADInstruments, Australia). Continuous finger arterial BP waveform was recorded through Finometer Pro (FMS, Amsterdam, Netherlands) that was adjusted against the brachial cuff BP. The pressure signal was fed to the PowerLab for recording. After a stabilization rest period of 5 minutes, baseline recording was done for 5 minutes.
Analysis of HRV was done through the software LabChart Pro (V. 8.1.13) and HRV module. The following HRV parameters were analyzed in time-domain: SDRR (standard deviation RR intervals) reflecting overall HRV, RMSSD (root mean square of successive differences of RR intervals), and pRR50 (percentage of successive RR intervals that are different by at least 50 msec). Both RMSSD and pRR50 provide information about parasympathetic function. The frequency domain parameters that were analyzed included total power (TP), which represents the overall total HRV, low-frequency (LF) and very low-frequency (VLF) bands indicating the sympathetic activity, high-frequency band (HF) to reflect parasympathetic activity, and LF/HF ratio to demonstrate the sympathetic-parasympathetic balance.12 Frequency domain HRV parameters LF and HF were computed both as absolute values (ms2) and in normalized units. To control for the possible confounding effect of respiration on HRV parameters, respiratory rate was measured via a respiratory belt.22
Cardiovascular Reflex Tests (CART)
Heart rate response to deep breathing, Valsalva maneuver and head-up tilt (HUT) were used to assess the parasympathetic function. Diastolic blood pressure responses to HUT and sustained isometric handgrip (IHG) were used to assess the sympathetic function.
Changes in Heart Rate During Deep Breathing (DB)
Participants were asked to complete six respirations in one minute under guidance of the examiner, whereby they had to inhale deeply for 5 seconds and exhale fully for 5 seconds in a smooth and continuous manner completing one respiratory cycle in 10 seconds. The differences between the highest and lowest HR during deep breathing was calculated. In addition, the ratio of maximum RR interval during expiration to minimum RR interval during inspiration (E:I ratio) was also calculated.10,11
Changes in Heart Rate During Valsalva Maneuver (VM)
The participants were instructed to exhale into a large dial aneroid sphygmomanometer, and were coached to keep the pressure at 40 mmHg for 15 seconds. The maneuver was performed thrice by every participant, with an intervening rest period of 2 minutes. The longest RR interval in the Phase IV and the shortest RR interval during the late Phase II of VM were identified from the ECG recording to calculate the Valsalva ratio.10,11
Heart-Rate Response to Head-Up Tilt (HUT)
After a resting period of lying down in supine position for 5 minutes, the table was tilted to 70 degrees and maintained for 5 minutes in this position. The table was tilted back and remained in supine position for another 5 minutes (Figure 1). The change in the heart rate was expressed as a ratio of the fastest heart rate (shortest RR interval) around the 15th beat to the slowest HR (longest RR interval) around the 30th beat after the head-up tilt.10,11
Changes in Blood Pressure During Head-Up Tilt (HUT)
Systolic and diastolic blood pressures were noted in the supine position as baseline measurements. Readings were taken again after 1–2 minutes after the tilt at 70 degrees (Figure 1).25,26
Changes in Blood Pressure During the Handgrip Test
After determining the maximum voluntary contraction with isometric force transducer, the participants were instructed to maintain the isometric handgrip for 3 minutes, during which the blood pressure was continuously recorded.10,11
Criteria for Grading Autonomic Function
An HR variation equal to or greater than 15 bpm or an expiratory/inspiratory ratio (E:I) of greater than or equal to 1.21 during DB were taken as normal. Similarly, a Valsalva ratio (VR) of equal to or greater than 1.21 was taken as normal. An HR response in the form of 30:15 R-R ratio of equal to or greater than 1.04 to HUT was taken as normal. An increase of DBP equal to or greater than 10 mmHg in response to sustained IHG was considered normal. Either no drop or a drop of less than 20 mmHg in SBP and/or a drop of less than 10 mmHg in DBP in response to HUT at 70 degrees tilt within 2 minutes were taken as normal. Any fall in SBP or DBP in response to HUT greater than the above-mentioned values were taken as postural or orthostatic hypotension (OH).27,28 Postural orthostatic tachycardia syndrome (POTS) was diagnosed if patients had an HR increase of 30 beats per minute (bpm) or HR above 120 bpm following the HUT in the absence of orthostatic hypotension.29 Results of CART were labeled as normal if no abnormal findings were detected, with parasympathetic dysfunction if 2 out of the 3 tests of the parasympathetic component were abnormal, with sympathetic dysfunction when 1 of the 2 tests of the sympathetic component test was abnormal, and with combined dysfunction when there is 1 abnormal test from each domain.30,31
Measurement of Cardiac Baroreflex Sensitivity (cBRS)
Cardiac baroreflex sensitivity (cBRS) is used as an index to evaluate the autonomic nervous system function. A reduction in the cBRS indicates cardiac autonomic dysfunction.32,33 Cardiac BRS was calculated offline by noting the instantaneous changes in heart rate in response to spontaneous changes in arterial BP with software PRVBRS provided by FMS (The Netherlands) using cross-correlation method.34 The correlation between beat to beat systolic BP and inter-beat interval was measured in a sliding 10-s window, with delays of 0 to 5 s for interval. The program selects the delay with the greatest significant positive correlation and the slope and the delay are recorded as one BRS value. BRS readings were averaged over at least 2–5 min except in deep breathing, where the maneuver itself was for 1 min only.34,35 The BRS data was displayed and analyzed with dedicated Beatscope® software version 1.1a. The inbuilt return-to-flow and height correction features enhanced the reliability and accuracy of Finometer recordings.36
Statistical Analysis
Data were presented as mean ± standard deviation (SD), median with interquartile range (IQ), or number (percent) where appropriate. Distribution of the data was tested using Shapiro–Wilk test of normality. Comparisons between groups were done using unpaired t-test and Mann–Whitney U-test for normal and non-normal distributed variables, respectively. Proportions were compared using the chi-square test. Comparison of the percent changes of different study variables between groups was done using ANCOVA with the baseline value as a covariate. Data was analyzed using SPSS 28.0 software; a P-value of <0.05 was considered significant.
Results
Fifty-nine subjects participated in this study. Both groups were matched in age (p=0.88), gender (p=0.99), and BMI (p=0.14). There were non-significant differences in the baseline heart rate (p=0.28), respiratory rate (p= 0.74), SBP (p=0.93), and DBP (p=0.66) between control and post-COVID groups. The median follow-up time of post-COVID subjects was 24 weeks (range 3–8 months). All subjects in both groups were vaccinated and without any comorbidities. The severity of illness among post-COVID group revealed 19 (68%) with mild and 9 (32%) with moderate acute illness based on the National Institute of Health (NIH) classification.37 (Table 1).
![]() |
Table 1 Demographic and Baseline Characteristics of Study Population |
Heart Rate Variability (HRV) and Cardiac Baroreceptor Sensitivity (cBRS)
Heart rate variability measurements (TP, LF, HF, LF/HF, LFnu, SDRR, RMSSD, and pRR50) were low in the post-COVID group, although statistically non-significant. Similarly, the cBRS measurements showed lower values in the post-COVID group, but did not reach a level of significance (Table 2).
![]() |
Table 2 Comparison of HRV Measurements and cBRS Between Groups |
Cardiovascular Reflex Tests (CART)
Orthostatic hypotension (OH) was demonstrated in 39.3% of post-COVID-19 participants in comparison to 3.2% of the control subjects, (p<0.001). Similarly, significant abnormal blood pressure response to the handgrip test was observed in the post-COVID group compared to the controls (73.1% vs 16.1%, respectively, p <0.001). Additionally, abnormal heart-rate response to HUT was higher in the post-COVID group (35.7%) compared to 12.9% in the controls (p=0.04) (Table 3). However, none of our subjects fulfilled the postural tachycardia syndrome (POTS) diagnosis criteria.
![]() |
Table 3 Comparison of Abnormal CART Results in Post-COVID Patients Compared to Control Group |
Isolated sympathetic dysfunction was reported in most post-COVID participants (71.4%) compared to controls (16.1%), (p <0.001); no isolated parasympathetic dysfunction was demonstrated in either group. However, a combined autonomic dysfunction was reported in 7.1% of post-COVID patients (Table 4). Cumulatively, about 85.7% of the post-COVID patients had at least one abnormal CART test in comparison with 35.5% within the control group (p <0.001) (data not shown).
![]() |
Table 4 Distribution of Sympathetic, Parasympathetic, and Combined Autonomic Dysfunction Between Groups |
Changes in Blood Pressure and Heart Rate Measurements During HUT
Both systolic and diastolic blood pressure showed a significant decrease from the baseline value after the HUT compared to the corresponding increase observed in the control group (p <0.001). Heart rate showed an increase during HUT in both groups, without significant difference (p=0.06) (Table 5).
![]() |
Table 5 Comparison of % Change in Systolic Blood Pressure, Diastolic Blood Pressure, and Heart Rate During Head-Up Tilting (HUT) |
Discussion
In the present study, the post-COVID group showed evidence of dysautonomia indicated by sympathetic dysfunction in response to cardiovascular challenges, thus suggesting changes in the autonomic control of cardiac function. Although the baseline HRV parameters and cardiac BRS were numerically lower in post-COVID group, this did not reach statistical significance. The CARTs demonstrated altered autonomic reactivity in some tests. There was a higher incidence of orthostatic hypotension in post-COVID patients compared to controls, and there was a significantly reduced diastolic blood pressure response to isometric handgrip test. Although the post-COVID group showed significantly abnormal heart rate response to head-up tilt, none of them fulfilled the postural tachycardia syndrome (POTS) diagnosis criteria.
Autonomic dysfunction has been described following several viral infections.14 HIV infection is associated with a reduction in the heart rate variability, and several autonomic manifestations including urinary system, gastrointestinal, secretomotor, pupillomotor, sleep, and male sexual function.38 Orthostatic hypotension and urinary dysfunction have been also described in mumps.39 Varicella zoster reactivation from autonomic ganglia could cause intestinal pseudo-obstruction. Rabies could also cause excessive salivation, piloerection, and photophobia. Furthermore, autonomic dysfunction may happen in acute viral encephalitis, herpes simplex, infectious mononucleosis, rubella, and coxsackie B virus.14
Both acute and delayed neurologic manifestations have been reported after SARS-CoV-2 infection. The receptors of SARS-CoV-2 are expressed in the central nervous system. The virus could spread directly through the cribriform plate and olfactory bulb, or through trans-synaptic invasion. Encephalitis, demyelination, neuropathy, and stroke are known complications of COVID-19.40 Additionally, autonomic dysfunction has emerged as a complication of COVID-19 infection; several case reports and observational studies revealed dysautonomia in association with SARS-CoV-2 infection.15,41 Dysautonomia in COVID-19 patients may manifest as labile blood pressure, postural hypotension, bladder dysfunction, gastrointestinal dysfunction, and impotence.42 The mechanisms of COVID-19-related dysautonomia are complex. SARS-CoV-2 can cause direct autonomic tissue damage, exaggerated immune response (innate and adaptive), and inflammation.43 During the cytokine storm, sympathetic stimulation induces the release of pro-inflammatory mediators, while parasympathetic activation elicits an anti-inflammatory response. Furthermore, an association between dysautonomia and the neurotropism of SARS-CoV-2 has been reported.44
Assessment of cardiac autonomic function can be carried out by specific tests and maneuvers on the cardiac sympathovagal system. Cardiovascular reflex tests (CART) involve a group of maneuvers that test both components of ANS (sympathetic and parasympathetic) separately.10 The current study reported postural hypotension in 39.3% of the post-COVID group during the blood pressure response to head-up tilt maneuver. Additionally, abnormal blood pressure response to the handgrip test was observed in about 73.1% of post-COVID patients. These two CART components reflected an impairment of the cardiac sympathetic function. Parasympathetic cardiac activity was also affected, as 35.7% of post-COVID patients showed abnormal heart rate response to the head-up tilt procedure. However, no postural tachycardia syndrome (POTS) was found in our cohort. Similar findings were reported by a recent study that included 180 post-COVID patients. Subjects were evaluated by active stand test between 4Â weeks and 9Â months from COVID-19 onset and orthostatic hypotension (OH) was diagnosed in 13.8% of the patients; none showed postural tachycardia syndrome (POTS).17 Another recent study in young adult post-COVID patients showed sympathetic over-activity and lower values of parasympathetic activity as evaluated by HRV measurement; these changes were modulated by body mass index (BMI).45 Furthermore, a study by Marques et al revealed a reduction in HRV with increased sympathetic modulation, and a decrease in parasympathetic modulation in long COVID.46 Cardiac autonomic dysfunction has also been reported during the early stage of COVID-19 diseases. Milovanovic et al showed sympathetic dysfunction with orthostatic hypotension in about 46.3%, and abnormal handgrip tests in about 94.4% of post-COVID patients. In addition, parasympathetic dysfunction was illustrated by abnormal heart rate response to the Valsalva maneuver and deep breathing.22
HRV is a tool that is commonly used to assess sympathetic and parasympathetic modulation of the autonomic nervous system, and it is a significant marker of dysautonomia.47 HRV is composed of a low-frequency band (LF), high-frequency band (HF), and very low-frequency band (VLF). The sympathetic and parasympathetic activity of the heart is reflected by LF, and considered an indicator of sympathovagal balance. HF assesses the parasympathetic activity of the heart, reflecting the vagal-mediated modulation.12 In our study, we found a non-significant reduction in TP, LF, HF, LF/HF, LFnu, SDRR, RMSSD, and pRR50 in the COVID-19 group. In contrast, a recent study involved 50 post-acute COVID subjects 20 weeks after recovery and found a decrease in the time domain measurements (SDNN, SDANN, SDNNi, RMSSD, pNN50) and frequency domain measurements (TP, VLF, LF, HF, and HFnu) in the post-acute COVID group compared to control subjects.48 Additionally, Milovanovic et al found significantly lower results in HF, and LF in COVID-19 patients during the early phase of COVID-19 infection.22 Furthermore, another study showed orthostatic hypotension in 13.04%, and POTS in 2.17%; heart rate variability (RMSSD) was significantly lower in post-COVID-19 patients compared to healthy controls (p=0.01).21
Body mass and level of physical activity were found to affect the autonomic function of post-COVID-19 patients; higher BMI post-COVID subjects demonstrated more dysautonomia in comparison with normal BMI controls. In addition, physically inactive post-COVID participants revealed more autonomic dysfunction compared to active controls.45 These results showed that dysautonomia associated with COVID-19 is potentially influenced by level of physical activity and BMI. Since post-COVID patients in the current study had almost normal BMI, this might explain why the observed reduction in HRV was not significant.
Baroreceptor sensitivity is crucial in assessing cardiac autonomic nervous function. It is measured by analyzing the spontaneous beat-to-beat changes of arterial blood pressure and heart rate; a reduction in BRS indicates dysautonomia.32,33 In our study, we showed a non-significant decrease of baroreceptor sensitivity in the post-COVID-19 group. In contrast, another study reported a significant reduction in mean baroreceptor sensitivity during the early phase of post-COVID-19 infection.22 This difference could be attributed to the difference in the time of autonomic function evaluation of post-COVID patients; the current study evaluated the post-acute effect post-COVID infection, while Milovanovic et al studied a group of active COVID-19 infections. This is in line with the finding that dysautonomia is more obvious following the acute stage of the viral illness,39,41 and could improve in time, either spontaneously or with treatment.49 In a recent study, heart rate recovery (HRR) following exercise cessation improved significantly 6 months after COVID infection.50 In addition, many factors could affect the development of dysautonomia following COVID-19 infection, including BMI, level of physical activity,45 and degree of inflammatory response.43
Limitations
Due to the cross-sectional design, it was difficult to conclude that a causal relationship exists between COVID-19 and dysautonomia. Additionally, the local restrictions of the COVID-19 pandemic made it difficult to recruit more subjects, which resulted in a relatively small sample size and may explain the non-statistically significant null findings of HRV and cBRS reported by this study. However, our results provide additional insights into the extent of cardiac autonomic dysfunction post-COVID-19 in a relatively young population.
Conclusion
The results of the present study are suggestive of altered cardiovascular reactivity as a post-acute sequela of COVID-19 infection, with a pronounced incidence of postural hypotension. However, this finding still needs future studies with a larger sample size investigating the mechanism of ANS involvement during the active infection as well as after COVID-19 recovery.
Abbreviations
LFnu, low frequency normalized unit; HFnu, high frequency normalized unit; TP, total power; LF, low frequency; HF, high frequency; LF/HF, low frequency/high frequency ratio; SDRR, standard deviation of RR intervals; RMSSD, root mean square of successive RR interval differences; pRR50, percentage of successive RR intervals that differ by more than 50 ms; cBRS, cardiac baroreceptor sensitivity; CART, cardiovascular reflex test.
Funding
There is no funding to report.
Disclosure
The authors report no conflicts of interest in this work.
References
1. Zhu N, Zhang D, Wang W., et al. A Novel Coronavirus from Patients with Pneumonia in China, 2019. N Eng J Med. 2020;382(8):727–733. doi:10.1056/NEJMoa2001017
2. Dashboard WCC. WHO Coronavirus (COVID-19) Dashboard With Vaccination Data [Internet]. 2022.
3. Michelen M, Manoharan L, Elkheir N, et al. Characterising long COVID: a living systematic review. BMJ Global Health. 2021;6(9):e005427. doi:10.1136/bmjgh-2021-005427
4. Zimmermann P, Pittet LF, Curtis N. The Challenge of Studying Long COVID: an Updated Review. Pediatr Infect Dis J. 2022;41(5):424–426. doi:10.1097/INF.0000000000003502
5. Deer RR, Rock MA, Vasilevsky N, et al. Characterizing Long COVID: deep Phenotype of a Complex Condition. eBioMedicine. 2021;1:74.
6. Raman B, Bluemke DA, Lüscher TF, Neubauer S. Long COVID: post-acute sequelae of COVID-19 with a cardiovascular focus. Eur Heart J. 2022;43(11):1157–1172. doi:10.1093/eurheartj/ehac031
7. Yan Z, Yang M, Lai CL. Long COVID-19 Syndrome: a Comprehensive Review of Its Effect on Various Organ Systems and Recommendation on Rehabilitation Plans. Biomedicines. 2021;9(8):966. doi:10.3390/biomedicines9080966
8. Long B, Brady WJ, Koyfman A, Gottlieb M. Cardiovascular complications in COVID-19. Am J Emerg Med. 2020;38(7):1504–1507. doi:10.1016/j.ajem.2020.04.048
9. Manolis AA, Manolis TA, Apostolopoulos EJ, Apostolaki NE, Melita H, Manolis AS. The role of the autonomic nervous system in cardiac arrhythmias: the neuro-cardiac axis, more foe than friend? Trends Cardiovasc Med. 2021;31(5):290–302. doi:10.1016/j.tcm.2020.04.011
10. Freeman R, Chapleau MW. Testing the autonomic nervous system. Handb Clin Neurol. 2013;115:115–136.
11. Zygmunt A, Stanczyk J. Methods of evaluation of autonomic nervous system function. Arch Med Sci. 2010;6(1):11–18. doi:10.5114/aoms.2010.13500
12. Camm AJ, Malik M, Bigger JT. Heart rate variability: standards of measurement, physiological interpretation and clinical use. Task Force of the European Society of Cardiology and the North American Society of Pacing and Electrophysiology. Circulation. 1996;93(5):1043–1065. doi:10.1161/01.CIR.93.5.1043
13. Goldberger JJ, Arora R, Buckley U, Shivkumar K. Autonomic Nervous System Dysfunction: JACC Focus Seminar. J Am Coll Cardiol. 2019;73(10):1189–1206. doi:10.1016/j.jacc.2018.12.064
14. Carod-Artal FJ. Infectious diseases causing autonomic dysfunction. Clin Auton Res. 2018;28(1):67–81. doi:10.1007/s10286-017-0452-4
15. Dani M, Dirksen A, Taraborrelli P, et al. Autonomic dysfunction in ‘long COVID’: rationale, physiology and management strategies. Clin Med. 2021;21(1):e63–e7. doi:10.7861/clinmed.2020-0896
16. Zirpe KG, Dixit S, Kulkarni AP, et al. Pathophysiological Mechanisms and Neurological Manifestations in COVID-19. Indian J Crit Care Med. 2020;24(10):975–980. doi:10.5005/jp-journals-10071-23592
17. Buoite Stella A, Furlanis G, Frezza NA, Valentinotti R, Ajcevic M, Manganotti P. Autonomic dysfunction in post-COVID patients with and without neurological symptoms: a prospective multidomain observational study. J Neurol. 2022;269(2):587–596. doi:10.1007/s00415-021-10735-y
18. Eldokla AM, Mohamed-Hussein AA, Fouad AM, et al. Prevalence and patterns of symptoms of dysautonomia in patients with long-COVID syndrome: a cross-sectional study. Ann Clin Translational Neurol. 2022;9(6):778–785. doi:10.1002/acn3.51557
19. Adler TE, Norcliffe-Kaufmann L, Condos R, et al. Heart Rate Variability Is Reduced 3- and 6-Months after Hospitalization for Covid-19 Infection. J Am Coll Cardiol. 2021;77(18):3062. doi:10.1016/S0735-1097(21)04417-X
20. Asarcikli LD, Hayiroglu M, Osken A, Keskin K, Kolak Z, Aksu T. Heart rate variability and cardiac autonomic functions in post-COVID period. J Interv Card Electrophysiol. 2022;63(3):715–721. doi:10.1007/s10840-022-01138-8
21. Shah B, Kunal S, Bansal A, et al. Heart rate variability as a marker of cardiovascular dysautonomia in post-COVID-19 syndrome using artificial intelligence. Indian Pacing Electrophysiol J. 2022;22(2):70–76. doi:10.1016/j.ipej.2022.01.004
22. Milovanovic B, Djajic V, Bajic D, et al. Assessment of Autonomic Nervous System Dysfunction in the Early Phase of Infection With SARS-CoV-2 Virus. Front Neurosci. 2021;2:15.
23. Hanson KE, Caliendo AM, Arias CA, et al. The Infectious Diseases Society of America Guidelines on the Diagnosis of COVID-19: molecular Diagnostic Testing. Clin Infect Dis. 2021;22:10.
24. Association WM. World Medical Association Declaration of Helsinki: ethical Principles for Medical Research Involving Human Subjects. JAMA. 2013;310(20):2191–2194. doi:10.1001/jama.2013.281053
25. Khemani P, Mehdirad AA. Cardiovascular Disorders Mediated by Autonomic Nervous System Dysfunction. Cardiol Rev. 2020;28(2):65–72. doi:10.1097/CRD.0000000000000280
26. Lahrmann H, Cortelli P, Hilz M, Mathias CJ, Struhal W, Tassinari M. EFNS guidelines on the diagnosis and management of orthostatic hypotension. Eur J Neurol. 2006;13(9):930–936. doi:10.1111/j.1468-1331.2006.01512.x
27. Novak P. Quantitative autonomic testing. J Vis Exp. 2011;19:53.
28. Hilz MJ, Dütsch M. Quantitative studies of autonomic function. Muscle Nerve. 2006;33(1):6–20. doi:10.1002/mus.20365
29. Raj SR, Guzman JC, Harvey P, et al. Canadian Cardiovascular Society Position Statement on Postural Orthostatic Tachycardia Syndrome (POTS) and Related Disorders of Chronic Orthostatic Intolerance. Can J Cardiol. 2020;36(3):357–372. doi:10.1016/j.cjca.2019.12.024
30. Jyotsna VP, Sahoo A, Sreenivas V, Deepak KK. Prevalence and pattern of cardiac autonomic dysfunction in newly detected type 2 diabetes mellitus. Diabetes Res Clin Pract. 2009;83(1):83–88. doi:10.1016/j.diabres.2008.09.054
31. Ewing DJ, Clarke BF. Diagnosis and management of diabetic autonomic neuropathy. Br Med J. 1982;285(6346):916–918. doi:10.1136/bmj.285.6346.916
32. Swenne CA. Baroreflex sensitivity: mechanisms and measurement. Neth Heart J. 2013;21(2):58–60. doi:10.1007/s12471-012-0346-y
33. Pinna GD, Maestri R, La Rovere MT. Assessment of baroreflex sensitivity from spontaneous oscillations of blood pressure and heart rate: proven clinical value? Physiol Meas. 2015;36(4):741–753. doi:10.1088/0967-3334/36/4/741
34. Westerhof BE, Gisolf J, Stok WJ, Wesseling KH, Karemaker JM. Time-domain cross-correlation baroreflex sensitivity: performance on the EUROBAVAR data set. J Hypertens. 2004;22(7):1371–1380. doi:10.1097/01.hjh.0000125439.28861.ed
35. Wesseling KH, Karemaker JM, Castiglioni P, et al. Validity and variability of xBRS: instantaneous cardiac baroreflex sensitivity. Physiol Rep. 2017;5(22):13509. doi:10.14814/phy2.13509
36. Guelen I, Westerhof BE, van der Sar GL, et al. Validation of brachial artery pressure reconstruction from finger arterial pressure. J Hypertens. 2008;26(7):1321–1327. doi:10.1097/HJH.0b013e3282fe1d28
37. COVID-19 Treatment Guidelines Panel. Coronavirus Disease 2019 (COVID-19) Treatment Guidelines. National Institutes of Health. Available from: https://www.covid19treatmentguidelines.nih.gov/.
38. Chow D, Nakamoto BK, Sullivan K, et al. Symptoms of Autonomic Dysfunction in Human Immunodeficiency Virus. Open Forum Infect Dis. 2015;2(3):3. doi:10.1093/ofid/ofv103
39. Mathuranath PS, Duralpandian J, Kishore A. Acute dysautonomia following mumps. Neurol India. 1999;47(2):130–132.
40. Montalvan V, Lee J, Bueso T, De toledo J, Rivas K. Neurological manifestations of COVID-19 and other coronavirus infections: a systematic review. Clin Neurol Neurosurg. 2020;194(105921):15. doi:10.1016/j.clineuro.2020.105921
41. Bosco J, Titano R. Severe Post-COVID-19 dysautonomia: a case report. BMC Infect Dis. 2022;22(1):214. doi:10.1186/s12879-022-07181-0
42. Almqvist J, Granberg T, Tzortzakakis A, et al. Neurological manifestations of coronavirus infections – a systematic review. Ann Clin Transl Neurol. 2020;7(10):2057–2071. doi:10.1002/acn3.51166
43. Larsen NW, Stiles LE, Miglis MG. Preparing for the long-haul: autonomic complications of COVID-19. Auton Neurosci. 2021;235(102841):3. doi:10.1016/j.autneu.2021.102841
44. Becker RC. Autonomic dysfunction in SARS-COV-2 infection acute and long-term implications COVID-19 editor’s page series. J Thromb Thrombolysis. 2021;52(3):692–707. doi:10.1007/s11239-021-02549-6
45. Freire APCF, Lira FS, Morano A, et al. Role of Body Mass and Physical Activity in Autonomic Function Modulation on Post-COVID-19 Condition: an Observational Subanalysis of Fit-COVID Study. Int J Environ Res Public Health. 2022;19(4):2457. doi:10.3390/ijerph19042457
46. Marques KC, Silva CC, Trindade SDS, et al. Reduction of Cardiac Autonomic Modulation and Increased Sympathetic Activity by Heart Rate Variability in Patients With Long COVID. Front Cardiovasc Med. 2022;9:862001. doi:10.3389/fcvm.2022.862001
47. Electrophysiology TFotESoCtNASoP. Heart Rate Variability. Circulation. 1996;93(5):1043–1065.
48. Kurtoğlu E, Afsin A, Aktaş İ, Aktürk E, Kutlusoy E, Çağaşar Ö. Altered cardiac autonomic function after recovery from COVID-19. Ann Noninvasive Electrocardiol. 2022;27(1):24. doi:10.1111/anec.12916
49. Blitshteyn S, Whitelaw S. Postural orthostatic tachycardia syndrome (POTS) and other autonomic disorders after COVID-19 infection: a case series of 20 patients. Immunol Res. 2021;69(2):205–211. doi:10.1007/s12026-021-09185-5
50. Cassar MP, Tunnicliffe EM, Petousi N, et al. Symptom Persistence Despite Improvement in Cardiopulmonary Health – Insights from longitudinal CMR, CPET and lung function testing post-COVID-19. E Clin Med. 2021;41(101159):20. doi:10.1016/j.eclinm.2021.101159
Sources 2/ https://www.dovepress.com/post-acute-effect-of-sars-cov-2-infection-on-the-cardiac-autonomic-fun-peer-reviewed-fulltext-article-IJGM The mention sources can contact us to remove/changing this article |
What Are The Main Benefits Of Comparing Car Insurance Quotes Online
LOS ANGELES, CA / ACCESSWIRE / June 24, 2020, / Compare-autoinsurance.Org has launched a new blog post that presents the main benefits of comparing multiple car insurance quotes. For more info and free online quotes, please visit https://compare-autoinsurance.Org/the-advantages-of-comparing-prices-with-car-insurance-quotes-online/ The modern society has numerous technological advantages. One important advantage is the speed at which information is sent and received. With the help of the internet, the shopping habits of many persons have drastically changed. The car insurance industry hasn't remained untouched by these changes. On the internet, drivers can compare insurance prices and find out which sellers have the best offers. View photos The advantages of comparing online car insurance quotes are the following: Online quotes can be obtained from anywhere and at any time. Unlike physical insurance agencies, websites don't have a specific schedule and they are available at any time. Drivers that have busy working schedules, can compare quotes from anywhere and at any time, even at midnight. Multiple choices. Almost all insurance providers, no matter if they are well-known brands or just local insurers, have an online presence. Online quotes will allow policyholders the chance to discover multiple insurance companies and check their prices. Drivers are no longer required to get quotes from just a few known insurance companies. Also, local and regional insurers can provide lower insurance rates for the same services. Accurate insurance estimates. Online quotes can only be accurate if the customers provide accurate and real info about their car models and driving history. Lying about past driving incidents can make the price estimates to be lower, but when dealing with an insurance company lying to them is useless. Usually, insurance companies will do research about a potential customer before granting him coverage. Online quotes can be sorted easily. Although drivers are recommended to not choose a policy just based on its price, drivers can easily sort quotes by insurance price. Using brokerage websites will allow drivers to get quotes from multiple insurers, thus making the comparison faster and easier. For additional info, money-saving tips, and free car insurance quotes, visit https://compare-autoinsurance.Org/ Compare-autoinsurance.Org is an online provider of life, home, health, and auto insurance quotes. This website is unique because it does not simply stick to one kind of insurance provider, but brings the clients the best deals from many different online insurance carriers. In this way, clients have access to offers from multiple carriers all in one place: this website. On this site, customers have access to quotes for insurance plans from various agencies, such as local or nationwide agencies, brand names insurance companies, etc. "Online quotes can easily help drivers obtain better car insurance deals. All they have to do is to complete an online form with accurate and real info, then compare prices", said Russell Rabichev, Marketing Director of Internet Marketing Company. CONTACT: Company Name: Internet Marketing CompanyPerson for contact Name: Gurgu CPhone Number: (818) 359-3898Email: [email protected]: https://compare-autoinsurance.Org/ SOURCE: Compare-autoinsurance.Org View source version on accesswire.Com:https://www.Accesswire.Com/595055/What-Are-The-Main-Benefits-Of-Comparing-Car-Insurance-Quotes-Online View photos
to request, modification Contact us at Here or [email protected]